
Flavor Science: How We Taste Sweet, Sour, Salty, and More
Understanding Taste and Flavor
Colloquially, the words “taste” and “flavor” are often treated as synonyms. I’m just as likely to say “I love the taste of napalm in the morning” as I am to say “I love the flavor of napalm in the morning.” I’d be misquoting Apocalypse Now either way (and I’d clearly need a refresher on what napalm is if I thought it was edible), but both words otherwise get the meaning across. For this discussion, though, we’re going to be more particular in our word choice.
When discussing “taste” here, we’ll be referring only to the very small set of five sensations our tongues can detect: salty, sour, bitter, sweet, and umami. (There’s increasing evidence that we may also have specific receptors on our tongues for fat, which would make it the sixth—and arguably most delicious—taste.) When we say “flavor,” meanwhile, we’re referring to the overall sensory experience, which includes not just those five or six tastes but also the incredibly complex and varied dimension of aroma.
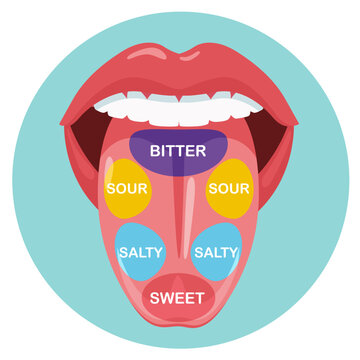
This common taste bud map is wrong. Keep reading to learn why.
For a lot of us, the thought of “taste” immediately conjures up one of those old taste bud maps. You know the ones—drawings of tongues with delineated regions, showing that we taste sour on the sides, sweet in the front, and bitter at the back. But that’s not really how it works. Instead, Crosby says, our tongues are covered in bumps, technically called “papillae,” and each papilla has thousands of taste buds on it. Each taste bud, in turn, contains roughly 100 taste cells. And each of those taste cells is designed to detect only one of each of the five (or six) tastes.* Despite what those taste bud maps have always shown us, the truth is that the cells are pretty well distributed all over the tongue.
There’s actually a very small amount of overlap in what the cells can detect, but for practical purposes, it’s easiest to think of each cell as being able to detect only one taste.
One of the most important things to understand about our ability to taste, according to Crosby, is that we’re genetically hardwired to do it. Coded into our DNA are the designs for very specific receptors to taste salt, acid, and the rest; each is there for the sole purpose of detecting those substances in our food and alerting our brains right away. Smell, on the other hand, is learned. “We don’t have a specific receptor for bacon smell,” Crosby says.
Instead, we use the roughly 400 different odor receptors in our nose to conjure up an impression of bacon. That doesn’t happen in the nose, though—it’s something our brains do. All in all, our brains can take the signals sent from those 400 olfactory receptors and assemble about 10,000 distinct smell sensations from them.
“Our sense of taste is fabricated right in our mouth,” Crosby told me. “Smells are fabricated in our brain.”
There are probably some very good evolutionary reasons for this. Our bodies need sodium chloride, what we commonly call salt, to regulate the amount of fluid in our cells and blood system; without it, we die. Sugar, meanwhile, is pure energy—our brains alone consume a quarter pound of it a day to keep running. Sourness signals not only spoilage (rotten foods often go sour) but also under-ripeness, which makes sense in light of how much we need sugar. Better to hold off on that tart peach and wait until it’s bursting with sugar before taking a bite. As much as some of us may think we can’t live without bacon, though, our DNA doesn’t appear to agree.
Interestingly, our sensitivity to each of the fundamental tastes is related to how much of them we do (or don’t) need. We need a lot of sugar, and so we’re the least sensitive to it; we’re about 10 times more sensitive to salt than sugar, causing us to consume less of it; umami, which indicates the presence of proteins, is roughly similar in this regard to salt; we’re 10 times more sensitive to sourness than to salt or umami; and then we’re at least 10 times more sensitive to bitter than to sour. Such high sensitivity to sour and bitter foods means that we end up more strictly limiting how much of them we eat—probably a good thing, given that they’re often signs of rot or poison. (Although, as any coffee addict, chocoholic, or beer guzzler can attest, it’s possible to override some of these aversions with a lifetime of dedicated training.)
And, of course, all of those signals are further complicated by the way our brain interprets them, which is dependent on past and current experience—your history with your own family recipes means that they taste different to you from how they do to a stranger, and a cold beer really does taste better on a hot day.
How We Experience Taste
Now that we’ve gone over the fundamentals of taste and flavor, let’s take a closer look at how our tongues detect each of these five (or, again, if we’re counting fat, six) tastes.
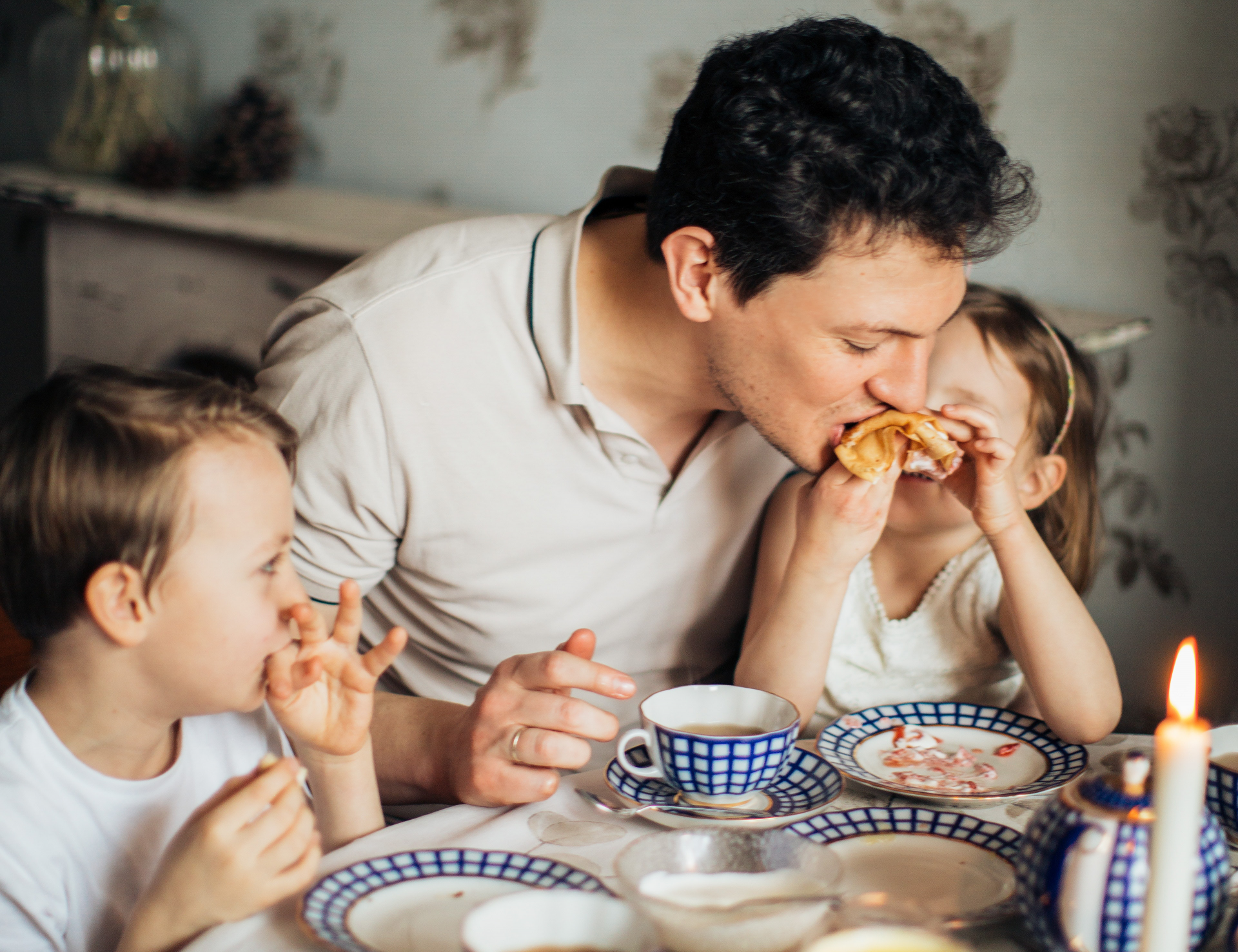
Our tongues detect the majority of the tastes—bitter, sweet, umami, and fat—using protein receptors on the surface of the taste cells. The receptors are like locks, and the bitter, sweet, umami, and fatty molecules are like keys: They snap together in specific ways, and when they do, the cells send signals to the brain reporting the molecules’ presence.
But salt and acid work differently. According to Crosby, our salt- and acid-detecting cells don’t have lock-and-key-like protein receptors on their surfaces. Instead, they have channels that allow salt and acid ions into the cells themselves. “Think of it like the Lincoln Tunnel,” he says. “They allow for the transport of ions from the outside of the cell, through the cell’s membrane, to the inside.” And, because ions are electrically charged, they change the electrical charge of the cells themselves, which tells the brain that it tastes salt or sourness.
This may not seem all that important, but it in fact holds at least part of the answer to why cooks and scientists use the words “acid” and “salt” differently.
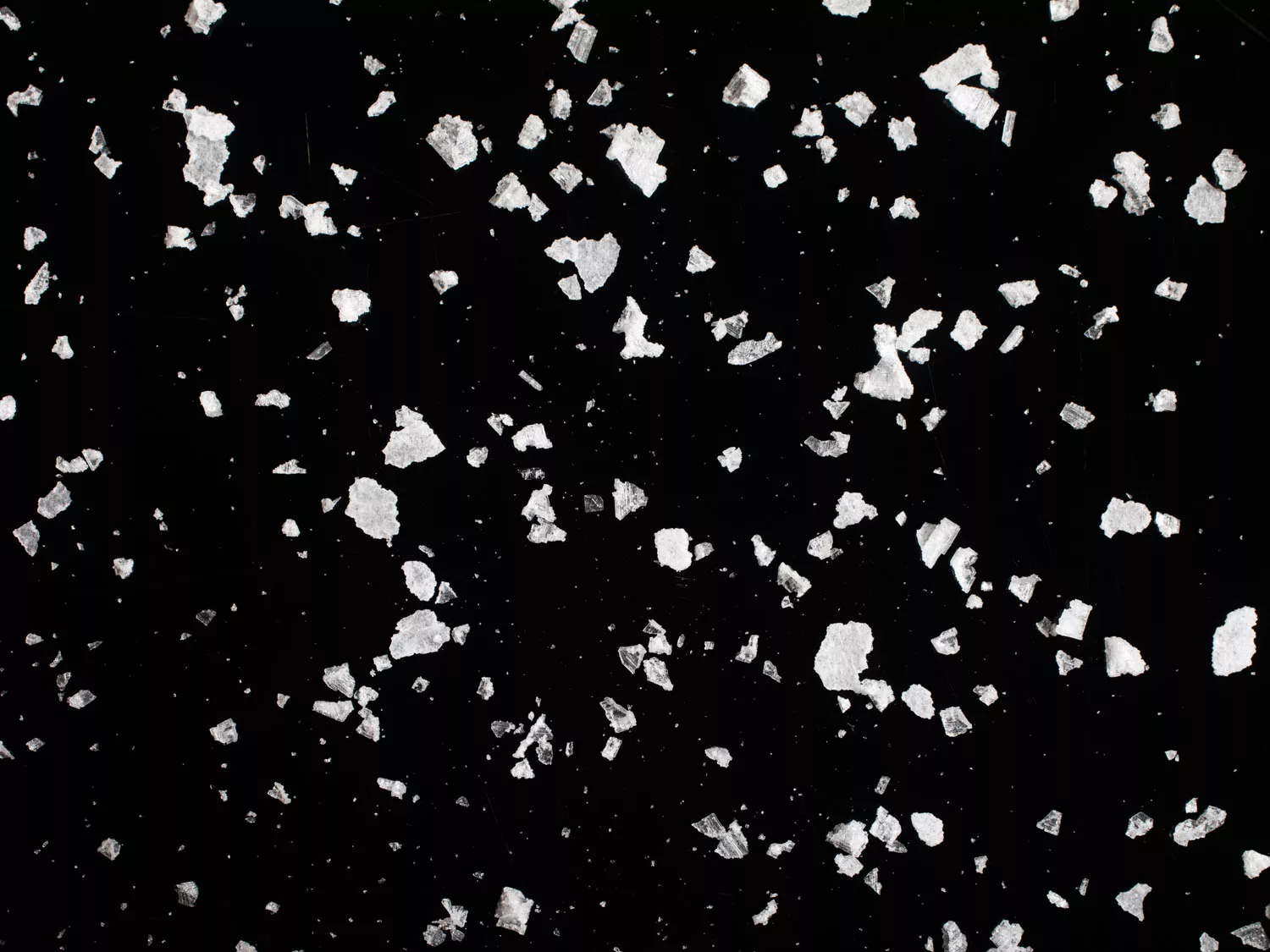
Salts and Acids: A Closer Look
A salt is a compound that results from the reaction of an acid with a base, and it breaks apart into both positive and negative ions when dissolved in water; those ions have positive and negative electrical charges (positive due to the absence of an electron, and negative due to an extra electron). So sodium chloride (NaCl), when dissolved in water, breaks apart into a positively charged sodium ion (Na+) and a negatively charged chloride ion (Cl-). All of the salt will always dissolve into the water until the water reaches its saturation point.
When it comes to tasting salt, the ion channels on our salt-sensitive taste cells are very small: just big enough to let the tiny sodium and chloride ions through, but not much else, including most other dissolved salts. This is the key to why sodium chloride is one of the only salts that actually taste salty to us. If we go back to Crosby’s Lincoln Tunnel analogy, it’s as if the tunnel were just large enough to let MINI Coopers and VW Bugs through, but anything sedan-size and up would smash against the entrance, never making it into the cell. Because there are so few salts that produce ions as small as NaCl does, it’s very difficult to make a convincing substitute for it—lithium chloride is one of the only ones that would work, but then we’d all be ingesting a powerful mood stabilizer as a seasoning on our eggs and potatoes. Potassium chloride comes with none of lithium’s side effects, but it has a noticeably bitter flavor that taints whatever it’s added to. (Some salt substitutes solve this by blending potassium chloride with sodium chloride to try to reduce sodium levels while minimizing that bitter taste, but it’s still not a dead ringer for our pure, beloved NaCl.)
Like salts, acids can also dissociate into positive and negative ions. In the case of acids, though, the positive ion is always hydrogen, and those hydrogen ions (also called protons) always produce a sour taste. The strength of an acid, however, is a function of not only its concentration but also its propensity to dissociate. Hydrochloric acid, for example, is very strong: Add it to water and almost 100% of it will break apart into positive hydrogen ions and their negative counterparts. If you were crazy enough to eat it (please don’t), it would be insanely sour, even in very dilute concentrations. Compare that to acetic acid (the acid in vinegar), of which only about 1% dissociates in water—and yet that’s still enough to make our mouths pucker. (Soy sauce, for reference’s sake, is about 10 times weaker as an acid than vinegar, based on their pH values.) The point here is that just because a food is technically acidic doesn’t mean that it will always have a strong, or even noticeable, sour flavor; it depends on the type of acid in question, and how concentrated it is.
Bringing This Back Into the Real World
This is all well and good, but before we sign off, it’s important to remember that the flavors of the food we eat are way more complicated than the types of isolated scientific examples here. “Pure science is different from the culinary applications because when you’re dealing with a food, you’re dealing with a complex mixture of things, as opposed to pure substances,” Crosby reminded me. As examples, he listed some of the ways in which the basic tastes can interfere with each other: Salt suppresses our perception of bitterness, and umami and acids enhance our perception of salt, while fat reduces our ability to taste salt. Then there’s the extra layer of aroma on top of that, which can influence our perception of whatever we’re eating in profound ways. “With soy sauce, it’s much weaker than vinegar as an acid, and then you have all these other components to it, like high levels of salt and glutamates,” he said. “There are so many powerful-tasting molecules in it, plus its aroma, they overwhelm any perception of sour taste.”
So, is soy sauce an acid? Well, technically, yes. Just…not in the kitchen.
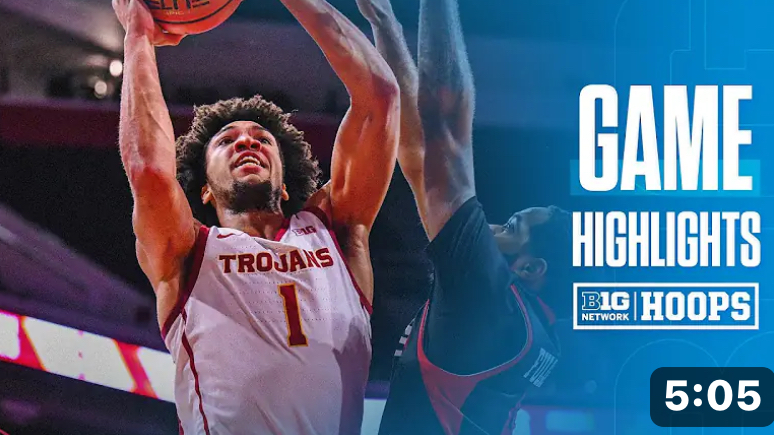
✌️ MENS 🏀 WIN!!

Hot Dog Grilled Cheese
